The wing skin, as an important part of the aircraft's aerodynamic structure, its performance quality is related to flight safety. The skin is equivalent to the "skin" of the aircraft, which is often used in the cross-sectional structures of the aircraft's wings, fuselage, etc. Damage to the skin can lead to the destruction of the aircraft's good aerodynamic performance, reduce the strength of the damaged area, and endanger flight safety.
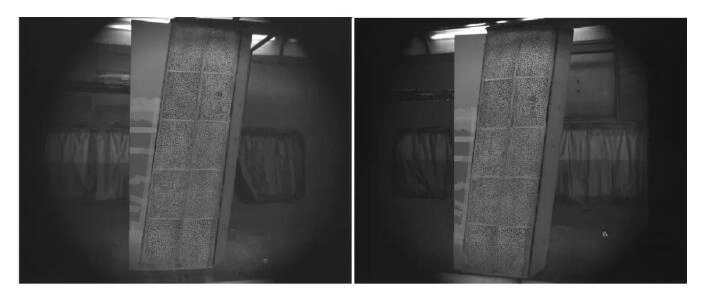
A research team from a certain university conducted a wind tunnel test on flexible aircraft skins. Using an optical strain measurement system, they studied the effects of angle of attack, wind speed, and temperature on the performance of the wing skin at a sweep angle of 7°. This investigation aimed to understand the mechanical characteristics of the wing structure under different working conditions and obtain results for out-of-plane displacement when changing a single variable among temperature, angle of attack, and wind speed (gathering modal parameters such as skin deformation and vibration information). The experiment provided important data for enhancing the performance of aircraft wings.
1. Experimental Process
Before the experiment began, the research team applied a layer of flexible material skin to the surface of the wing shell and secured it in place. They then created speckles on the outer surface of the flexible material skin. The wing was adjusted to a sweep angle of 7° and fixed onto a mount within the wind tunnel. Through the braking device, the wing's angle of attack was set at -4° and 0°, and a heating device was adhered inside the wing.
The experiment collects and records the initial state of the wing through the Revealer DIC measurement system, and segments record real-time data under three wind speeds, with a sweepback angle of 7° and attack angles of -4° and 0°. It also collects and records strain data at the same experimental angles when the flexible material skin reaches thermal equilibrium.
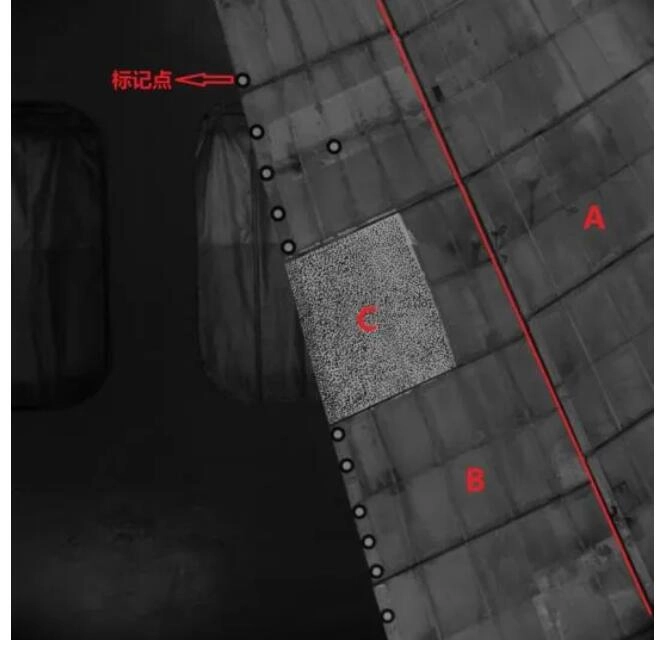
The experiment initiates the wind tunnel with the wind speed gradually increasing to 30m/s. Once the wind speed stabilizes, high-speed data collection is conducted; the wind speed slowly rises to 40m/s, and after stabilizing, high-speed data collection is performed again; the wind speed gradually increases to 45m/s, and after stabilizing, high-speed data collection is carried out. The experimental setup under heating thermal equilibrium state repeats the operations as described above.
2. Experimental Data Analysis
Since the high-speed acquisition process is carried out while the wing is in a stable state, during the initial stage, three frames of images collected under wind speeds of 30m/S, 40m/S, and 45m/S are selected for calculation. One frame from the initial stage is used as a reference image, while the other two frames are used to measure errors caused by environmental and system factors. One frame from each of the three wind speeds is selected for comparison with the frame from the initial stage.
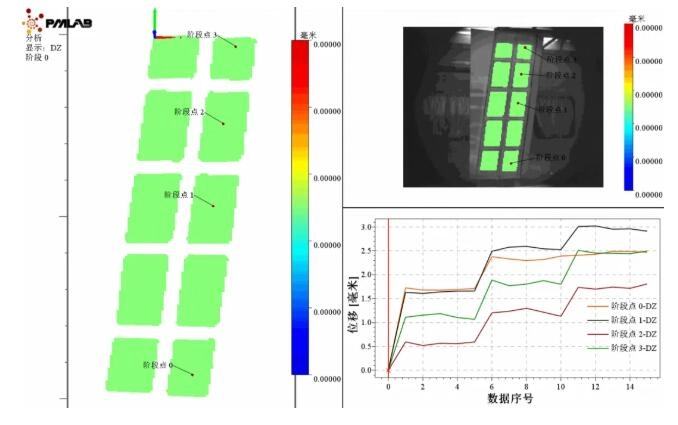
During data processing, a coordinate system is established by "selecting the origin" in the measurement system, resulting in a right-handed system with the symmetrical center of the wing surface at the initial state as the origin. The positive direction of x is downward along the longitudinal axis of the wing, and the positive direction of z is inward from the surface. A positive displacement from the surface indicates the wing is lowering, while a negative displacement indicates the wing is rising. Subsequently, rigid body displacements are removed from the data relative to the reference figure, except for those serving as references. Cloud maps are exported based on the grid points divided by regions, showing the displacement from the surface at each grid point, the maximum and minimum values of the overall displacement from the surface, and the results of the other two frames under three wind speeds can be compared with the selected frame as proof of the wing being in a stable state.
3. Experimental Results Analysis
① After the wind tunnel is opened, there will be bulges and depressions in the local area of the flexible material skin of the wing.
② The impact of angle of attack on the performance of flexible material skins of wings at a sweep angle of 7°.
Through the analysis of Tables 1 and 2, it is found that under the same conditions of temperature, wind speed, and sweep angle, when the attack angle is 0°, the standard deviation of off-surface displacement is the smallest, indicating that the material deformation is more uniform at this time; the minimum value of off-surface displacement is the smallest, indicating that the local bulging on the material surface is the least; the difference between the maximum and minimum off-surface displacement is the smallest, indicating that the maximum deformation on the material surface is smaller. Therefore, it can be concluded that the material performance is optimal when the attack angle is 0°.
③ Effect of temperature on the performance of flexible material skins for wings at a sweep angle of 7°.
Comparing and analyzing the data in Tables 1 and 2, it can be observed that under the same conditions of angle of attack, wind speed, and sweep angle, the standard deviation of surface displacement from the flexible material skins of the wings is larger in the heated thermal equilibrium state, indicating more uneven deformation of the material at this time; the maximum value of surface displacement is larger, suggesting that there are larger local bulges on the material surface; the difference between the maximum and minimum surface displacement values is larger, indicating a greater maximum deformation on the material surface. From this, it can be concluded that the material performs better at room temperature.
④ The influence of wind speed on the performance of flexible material skins for wings at a sweep angle of 7°.
By observing each column in Tables 1 and 2, it can be seen that when the temperature, attack angle, and sweep angle are the same, the smaller the wind speed, the smaller the standard deviation of off-plane displacement, indicating that the material deformation is more uniform at this time; the smaller the maximum value of off-plane displacement, indicating that the local bulge on the material surface is smaller; the smaller the difference between the maximum and minimum off-plane displacement, indicating that the maximum deformation amount on the material surface is smaller. It can thus be concluded that when the wind speed is 30 meters per second, the material performance is optimal.
Summary
Through flexible material skin testing in wind tunnels, we gain further understanding of wing skin performance to achieve various deformation detections of the wings, enhancing aircraft maneuverability to adapt to different environmental and mission-specific flight requirements. Aircraft manufacturing relies on the quality control of key components. To ensure flight safety, the design of each component and the strain testing of materials require advanced measurement and analysis methods, applying experimental data to optimize manufacturing designs.